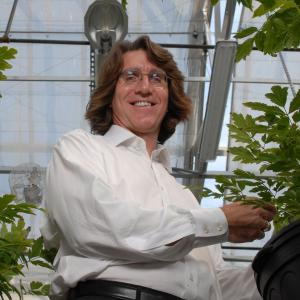
Contact
Email: eisenste@umd.edu
Call: (240) 314-6244
Edward Eisenstein
Associate Professor
Eisenstein Group
Contact
Email: eisenste@umd.edu
Call: (240) 314-6244
Education
- Postdoctoral Research, Molecular Biology, University of California Berkeley, 1985-1989
- Ph.D., Biochemistry, Georgetown University, 1985
- B.S., Biology and Chemistry, Saint Joseph’s College, 1979
Profile
The Eisenstein laboratory is building next-generation plants as robust and healthy feedstocks for the bioeconomy by enhancing their capacity to manage nutritional, biotic and abiotic challenges. Their strategy is to derive mechanistic information from multidisciplinary tools, ranging from molecular and structural biology, to plant, systems and synthetic biology, as well as protein design and engineering, to develop superior traits. These improvements are being introduced into poplar via genome engineering in order to expand the scope and scale of bioproducts from trees. In addition, Dr. Eisenstein is engaged in project-based learning opportunities for students as the founding co-advisor for the UMaryland iGEM team, introducing nearly 200 undergraduates to synthetic biology research aimed at solving global problems, enabling them to compete successfully at the yearly international Jamboree. Finally, the laboratory continues to apply systems engineering principles to improve the development of protein biopharmaceuticals.
FOUNDATIONS FOR CURRENT RESEARCH
The laboratory’s current research interests rest upon a two-decade foundation of broad, collaborative, and multidisciplinary research that utilized molecular, genetic, structural and biophysical approaches to investigate the architectures and mechanisms of interaction among biological macromolecules. Past work led to the discovery of a new molecular mechanism for allosteric control of bacterial branched chain amino acid biosynthesis (Eisenstein, J. Biol. Chem., 266: 5801, 1991; Eisenstein, Biochemistry 34: 9403, 1995; Gallagher, Structure 6, 465, 1998; Gallagher, Meth. Enzymol. 380, 85, 2004). Research on the elementary steps of the interaction of molecular chaperones with newly synthesized proteins generated new thinking about the ability of chaperones to unfold tangled polypeptides in the process of catalyzing intracellular protein folding (Lin, J. Biol. Chem., 270: 1011, 1995; Lin, PNAS 93: 1977, 1996). New experimental tools and technology were developed for the quantitative measurement of high-affinity protein interactions (Myszka, J. Biomolec. Techniq. 14, 247, 2003; Navratilova, Analytical Biochemistry 344, 295, 2005), stimulating several community-wide inter-comparability studies of biomolecular interactions to reveal weaknesses in common protocols and to train new users (Yamniuk, J. Biomolec. Techniq. 23, 101, 2012; Yamniuk, J. Biomolec. Techniq. 26: 125, 2015). Progress in these areas, as well as new experimental tools and technology developed for high-throughput approaches for the expression, purification and characterization of proteins, helped launch the very first funded structural genomics program to provide insight into the function of gene products of unknown function in Haemophilus influenzae by elucidating their structures (Eisenstein, Curr. Opinion in Biotechnology 11, 25, 2000), an effort that promoted an entire initiative at NIH. These activities led to the pursuit and elucidation of the structures and mechanisms of the biosynthesis of phenazine antibiotics in Pseudomonas species (Parsons, Biochemistry 42, 5684, 2003; Bera, Biochemistry 48, 8644, 2009), shedding new light on the ability of pathogenic organisms to communicate and to promote infection, and providing new strategies for drug development. In their initial pivot to studies on plants, the laboratory focused on the medicinal perennial Actaea racemosa (black cohosh), a botanical supplement for menopausal symptoms (Spiering, Meth. Molec. Biol. 1083, 253, 2013; Kaur, Amer. J. Plant Sci., 4, 77, 2013), with systems biology and biochemical studies revealing an unusual pathway for biogenic tryptamine biosynthesis (Spiering, Plants, 14: 292, 2025).
CURRENT RESEARCH
Poplar Trees as an Enhanced Feedstock for the Bioeconomy
A challenge to improving the quality and availability of plant feedstocks is that transgenic plants with enhanced biotechnology traits (such as enhanced yields of bioproducts) often display reduced fitness, lower biomass, and an increased susceptibility to disease. The Eisenstein lab focuses on poplar trees as a feedstock because they grow rapidly and produce significant biomass in short times. Poplar is also a good model for perennials thanks to an increasingly accessible set of genomic and experimental resources that make it an attractive system for improvement. The lab is building on profitable collaborative work that resulted in a high-throughput and high-efficiency approach to poplar transformation and genome engineering. The relative ease in generating large sets of transgenic poplar lines is enabling coupled molecular, systems and plant-based approaches to discover interconnected networks and develop next-gen trees for unprecedented biotechnology application. Three challenges to improving poplar as a robust plant feedstock are being addressed:
Increase plant resistance to pathogens
Plant pathogens use a variety of mechanisms to infect and spread disease, but the plant immune system limits infection by recognizing pathogen effectors and activating local cell death. Leaf rust – one of the most important diseases in poplar - is caused by the pathogenic fungus Melampsora larici-populina. The lab is elucidating the components of the poplar defense response that are targeted by rust effectors, as well as the components of poplar nutrient homeostasis that are hijacked by the pathogen to spread disease. Additionally, the lab is determining the structures of receptor-effector complexes to unravel the elementary steps of host-pathogen interactions. These outcomes will afford new approaches for genetically engineering useful biotechnology traits into poplar to enhance resistance to pathogens and expand its range and therefore potential for use.
Remobilize nutrients to increase biomass
Seasonal nitrogen cycling plays a key role in the overall nitrogen budget of poplar and has a significant impact on new growth and biomass yield. The lab is using protein, metabolic, and systems engineering to enhance the synthesis and storage of nitrogen-rich metabolites and to rewire the signaling between nitrogen utilization and storage to improve Nitrogen Use Efficiency (NUE). The outcomes will increase biomass yields and enhance efficient downstream processing to advance the long-term sustainability of poplar as a plant feedstock for bioproducts.
Improve plant response to abiotic stress
A barrier to the broad adoption of plant feedstocks is lignin – a phenolic-based polymer that provides rigidity and strength to plant tissues, but which must be removed from pulp to extract economically attractive products. Low lignin plants have displayed enhanced product yields, but are prone to metabolic stress since they cannot as readily cope with a host of abiotic challenges, especially constant exposure to UV radiation. Interestingly, transgenic poplar lines in which the expression of UVR8, the green plant photoreceptor for harmful UV-B radiation, display enhanced growth. Ecophysiologic, metabolomic and structure-function investigations of genetically engineered poplar and their receptor-protein interactions will help to decipher the mechanisms whereby low-lignin plants respond to UV stress, and how the UVR8 receptor influences growth. The results will inform engineering approaches to boost acclimation and fitness of poplar as an attractive resource for the bioeconomy.
Enhance Soluble Protein Production
The lab also has a long-standing interest in improving the production of therapeutic proteins in bacterial and mammalian cell cultures. They are using modern genome-engineering approaches to rewire cell circuits to improve the production of therapeutic proteins. Their goal is to establish cell lines that enhance soluble protein production for a range of therapeutic protein targets.