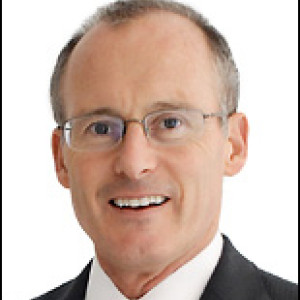
Contact
Email: gpayne@umd.edu
Call: (301) 405-8389
Gregory Payne
Research Professor
Payne Group
Contact
Email: gpayne@umd.edu
Call: (301) 405-8389
Education
- Ph.D., Chemical Engineering, The University of Michigan, 1984
- M.S., Chemical Engineering, Cornell University, 1981
- B.A., Chemical Engineering, Cornell University, 1979
Profile
The last century witnessed spectacular advances in both microelectronics and biotechnology yet there remains considerable opportunity to create synergies between the two. The Payne laboratory aims to fabricate high-performance material systems to span the capabilities of biology and information technology. Through an extensive network of international collaborations their group focuses on two primary areas of research:1) biofabrication of the bio-device interface and 2) redox-based molecular communication for bio-device "connectivity".
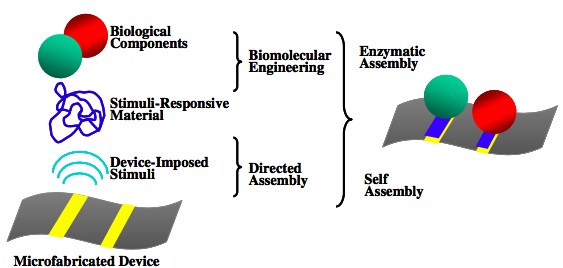
CURRENT RESEARCH
Biofabrication of the Bio-device Interface
Biology is expert at creating functional nanoscale components (e.g., proteins) and assembling them over a hierarchy of length scales. However, biology’s use of labile components and bottom-up assembly differs markedly from the “bio-incompatible” top-down methods used to fabricate microelectronic devices. Biofabrication – the use of biological materials and mechanisms for construction – offers the opportunity to span these divergent fabrication paradigms by providing convergent approaches for building the bio-device interface. Figure 1 illustrates the lab’s vision for biofabricating the bio-device interface using device-imposed electrical stimuli to provide the cues to trigger biopolymers (e.g., the polysaccharide chitosan) to locally self-assemble into a hydrogel matrix at an electrode address. Importantly, the electrodeposited biopolymer hydrogels provide the water-rich microenvironment that is compatible with biology. Further, these biopolymers can be bio-functionalized through biological mechanisms (e.g., enzymes can be used to graft functional components onto these biopolymer hydrogels).
Redox-based Molecular Communication for Bio-device "Connectivity"
Biology and electronics are each expert at processing information yet they use entirely different signaling modalities. Biology and electronics are each expert at processing information yet they use entirely different signaling mechanisms. Biology signals using ions and molecules, while modern devices use electrons to process information. Oxidation-reduction (i.e., redox) reactions provide the bridge to connect the molecular modalities of biology with the electronic modalities of devices. The Payne lab is developing new experimental methods (e.g., spectroelectrochemistry) to study redox-based communication. These methods are providing new insights on biological communication and suggest how redox-active biological materials such as melanin may interact with their environment (Figure 2).
The lab is also developing signal processing methods that enable devices and biology to “talk” to each other through redox mechanisms (Figure 3). Coupling these methods with advances in synthetic biology (synbio) promises to offer transformative capabilities by enabling the integration of the information processing capabilities of biology into electronics. Specifically, synbio constructs are being engineered to transduce molecular information into electrical outputs for advanced sensing, while communication in the opposite direction is enabling electrical inputs to be transduced by other synbio constructs into molecular outputs that can alter biological behaviors.
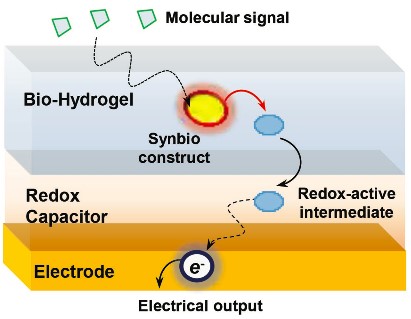
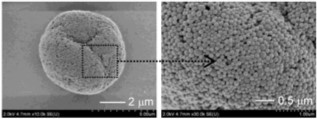